EDUCATION
CORNER
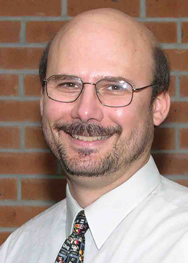
Robert Bateman (Robert.Bateman@usm.edu) is a Professor of Biochemistry at the University of Southern Mississippi, where he has been a faculty member since 1988. He holds a bachelor's degree in biochemistry from LSU and a doctorate in biochemistry from University of North Carolina at Chapel Hill. He performed postdoctoral work at the UT-Southwestern Medical Center in Dallas and did a sabbatical at Duke University with Jane and David Richardson in 1999. His website is ocean.otr.usm.edu/~w304739/.
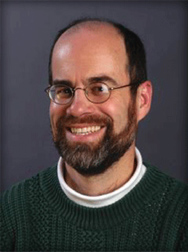
Paul Craig (Paul.Craig@rit.edu) a member of RCSB PDB Advisory Committee is a Professor of Biochemistry and Bioinformatics at the Rochester Institute of Technology, where he has been a faculty member since 1993. He holds a bachelor's degree in chemistry from Oral Roberts University and a doctorate in biological chemistry from the University of Michigan. His postdoctoral work at the Henry Ford Hospital in Detroit, MI was followed by a sabbatical at the San Diego Supercomputer Center with the RCSB PDB's Philip Bourne in 2002. His website is people.rit.edu/pac8612/.
A Proficiency Rubric for Biomacromolecular 3D Literacy
by Robert C. Bateman, Jr. and Paul A. Craig
Biochemistry educators use a burgeoning variety of tools to teach concepts in higher order molecular structure, but continue to struggle with how to assess the effectiveness of these tools and approaches in promoting student learning. Resolution of this issue is important if we are to compare studies of teaching effectiveness. As a step towards this, we are expanding on the idea of molecular 3D literacy1; 2 to propose a set of standards for achieving a level of proficiency in structural biology concepts appropriate to various educational levels. Such standards should not only provide a framework for assessment of teaching efficacy by novice and experienced instructors alike, but also enlighten developers of molecular visualization tools as they consider the education-oriented end-user.
Based on our own experiences over decades of teaching structural concepts in biochemistry,1-4 w
e considered several factors in developing such a set of standards for molecular 3D literacy. First, we framed the standards in terms of a one-page proficiency rubric. While there are undoubtedly those who would argue that it is too limiting, we believe that anything more comprehensive will simply not be useful to most instructors who use these standards as a basis for assessing the effectiveness of teaching structural concepts. Second, we divided the rubric into three columns to correlate proficiency levels with the appropriate educational objectives of the course. This makes it not only useful in a wide variety of educational settings ranging from high school to graduate school, but enables its direct use in assessing advanced courses. Third, the rubric should be as independent of the teaching modality and technology as possible, i.e., it needs to separate the concepts from the tools. It would therefore be valuable in assessing learning with any kind of molecular visualization tool including hard models, graphic rendering programs like Jmol5 and PyMOL6, simulations, animations, interactive games, haptics, etc. Fourth, the rubric is intentionally broad enough to cover a variety of biomacromolecules and is thus not limited to the usual protein structure concepts.
As we use different molecular visualization tools with students and demonstrate them to colleagues who teach at levels from secondary school through college, we find that their fascination with the beauty of the images and animations interferes with higher level thinking about the structures themselves. One of the goals of establishing the rubric is to introduce users to critical thinking concerning the 3D data they encounter. Some items in the rubric directly address this issue: atomic geometry and structural model skepticism. Other items gauge the user's ability to employ the molecular visualization software as something other than a black box: structure-function relationships, topology, and connectivity.
The proficiency rubric shown below has been reproduced from the original at ocean.otr.usm.edu/~w304739/MolVisProf.pdf. It is composed of a one-page grid followed by a one-page legend of the categories included. These categories address information in alternate renderings, molecular motion, structure-function relationships, limitations of molecular models, geometric constraints, recognition of higher order symmetry, chain topology, intermolecular interactions, monomer and het group recognition, and construction/annotation. This last category is not so much a concept as the ability to apply the other molecular concepts to a new situation.
Since this is intended to be a tool that will benefit everyone who teaches biomacromolecular structure concepts, we ask the community to assist in refining this tool by providing feedback directly to one of us and by using the rubric in your courses. If you develop a grading/assessment rubric of your own that is tailored to your purposes, please send us a copy along with information about your course and your contact information so we can properly acknowledge your contribution.
- R. C. Bateman Jr., D. Booth, R. Sirochman, J. Richardson & D. C. Richardson. (2002) Teaching and assessing three-dimensional molecular literacy in undergraduate biochemistry. J Chem Educ 79, 551.
- D. Booth, R. C. Bateman Jr., R. Sirochman, D. C. Richardson, J. S. Richardson, S. W. Weiner, F. M. & C. Putnam-Evans. (2005) Assessment of molecular construction in undergraduate biochemistry. J Chem Educ 82, 1854-1858.
- 13. L. Grell, C. Parkin, L. Slatest & P. A. Craig. (2007) EZ-Viz, a tool for simplifying molecular viewing in PyMOL. BAMBED 34, 402-407.
- 14. P. Yang, P. A. Craig, D. Goodsell & P. E. Bourne. (2003) BioEditor-simplifying macromolecular structure annotation. Bioinformatics 19, 897-898.
- Jmol: an open-source Java viewer for chemical structures in 3D. http://www.jmol.org/
- W. DeLano. (2002). The PyMOL molecular graphics system, http://www.pymol.org/
|